Madeline Lancaster opens the door of a fridge-sized incubator in her lab at the University of Cambridge's biomedical campus. On its gently gyrating shelves sit glass dishes containing a pinkish liquid. She takes one of them out to show what lies inside. Sitting in the nutrient fluid are half a dozen lumpy white blobs, roughly the size of large lentils.
“These are cerebral organoids, or mini-brains for short,” says Lancaster in a soft American voice. “They are three-dimensional neural tissues generated from human stem cells which allow us to model human brain development.”
“They are self-organising,” she adds. “We’re allowing these cells to develop on their own in a 3D conformation, which is the way the brain develops naturally.”
Viewing an organoid through Lancaster’s high-powered microscope its million or so neurons look like an entangled cellular web very similar to an embryonic human brain.
Lancaster pioneered the cerebral organoids field, making the world's first mini-brains in 2011 while working as a post-doctoral researcher at IMBA, Austria's Institute of Molecular Biotechnology in Vienna. She moved in 2015 to set up her own lab in Cambridge at the Medical Research Council's laboratory of molecular biology (LMB).
Today more than 100 labs worldwide are working on cerebral-organoid research, one of the fastest growing fields in biology. Scientists see organoids both as a route to understanding how the human brain develops and as a better way for the pharmaceutical industry to test the effect of drugs on the brain.
At the same time bioethicists are anticipating a possible future in which the technology advances to the point where the mini-brains begin to think.
“Although we are very, very far from sentient brain organoids, it is not too soon to consider the ethical side of this research,” says Lancaster. “Brain organoids are a powerful and much-needed tool for understanding how the human brain develops and how that process can go wrong. But we have to take into account the views of society and religion as well as scientists, so I am getting involved with the ethics too.”
Julian Savulescu, bioethics professor at Oxford University, praises her involvement. "We have a moral imperative to do organoid research because of its potential for curing disease," he says. "Currently there are no ethical problems with it but we can't assume that it will never be possible to produce potentially conscious brain material in the lab."
Lancaster grew up in Salt Lake City, the daughter of a psychiatrist mother and biochemist father.
"There was no doubt that I would go into science. I don't think they pushed me in that direction. I wanted to go to college in a big city and went to Los Angeles as an undergraduate at Occidental College. I was interested in psychology but wanted to find out what was actually happening in the brain when people were thinking."
Lancaster did her PhD at the University of California, San Diego, a leading centre for neuroscience research, and then moved further afield to IMBA in Vienna.
Embryos
There she joined the lab run by German molecular biologist Jürgen Knoblich to study how the brain develops in embryos. Knoblich was working mainly with Drosophila (fruit flies) as a model organism and wanted to use Lancaster’s expertise in mouse development to extend the research to mammalian systems.
Like many scientific discoveries, Lancaster’s brain organoids resulted from a fortuitous accident. She was trying to culture mouse neurons in a two-dimensional form known as neural rosettes.
“I was having problems getting them to attach to the dish. They started to form floating balls of tissue. To make them stick better I applied a coating of Matrigel, a gelatinous protein mixture. The cells went into the gel and began to form what we now call brain organoids.”
Hans Clevers at the University of Utrecht had made intestinal organoids – or mini-guts – from human stem cells in 2009, but Lancaster's were the first mini-brains. She and Knoblich agreed that she should focus her research on organoids and switch from mouse to human cells.
The work came to public attention when Lancaster and her IMBA colleagues published a landmark paper in Nature in 2013, accompanied by a press teleconference and a news release proclaiming “Human brain grown in lab”. Not surprisingly, the discovery received excited media coverage, with many publications picking up on the lab’s reference to “mini-brains”.
Many neuroscientists, including Knoblich, regret the use of the term “mini-brain”, which they say suggests a cognitive capacity far beyond today’s organoids. Lancaster herself has mixed feelings.
“Yes, the IMBA press release contained the phrase ‘mini-brain’. I can’t remember who first suggested it,” she says. “It’s not a term that everyone in the field likes – and I haven’t made my mind up about it – but I think the public is smart enough to know that they are not actually miniature brains.”
As a philosopher of science Savulescu also avoids “mini-brain” when talking about cerebral organoids. He says words really matter when people are introduced to potentially controversial new biotechnology. “For the general public the terminology sets the tone of the debate in a way that is hard to escape.”
Organoids might be useful for scientists seeking to understand and treat a wide range of neurological disorders from autism to epilepsy. An early example is microcephaly, a condition marked by very small brain size. Lancaster and colleagues made stem cells from the skin of microcephalic patients and from these grew cerebral organoids – which turned out to be abnormally small. The researchers then identified a defect in the neural development process in microcephaly, which could in principle be corrected through genetic manipulation.
Researchers
During her four years at the LMB in Cambridge, Lancaster’s research has moved in two main directions. One focuses on evolutionary neurobiology, using organoids to compare brain development in different species.
“We collaborate with zoos and with researchers who have already made stem cells from other species,” she says. “The cells often come from veterinary procedures on zoo animals, for example blood samples from chimps or a benign tumour removed from a gorilla.”
The other strand of her research is finding ways to make superior mini-brains. The original method produces organoids that stop growing after a few months, when they reach a diameter of about 5mm because they have no vasculature (blood supply) to bring nutrients to their inner cells, which therefore die.
Several innovations are beginning to overcome these limitations. An experiment at the LMB, published in March, cut a human organoid into thin slices, which received nutrients from the liquid in their dish and oxygen from the air. They remained healthy for a year and formed functional connections with a small section of spinal cord and associated muscles taken from mouse embryos. Electrical impulses from neurons in the organoid made the muscles contract.
“It was exciting to see the human neurons connecting with the mouse neurons,” Lancaster says.
A quite different human-mouse combination, published last year, took a step towards solving the vascularisation problem. Scientists at the Salk Institute in California transplanted human organoids into the brains of live mice. Murine blood vessels grew into the organoids, nourishing them and enabling them to expand, though behavioural tests provided no evidence that the additional human element boosted the mice's intelligence.
“The ability to achieve vascularisation and integration of brain organoids with a host is a major leap in studying the developing human brain within the context of a whole organism,” says Lancaster, who was not involved in the Salk project.
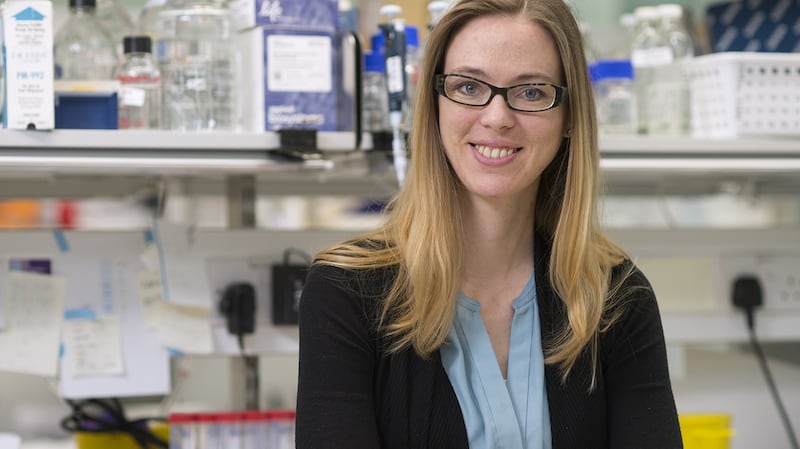
For Knoblich at IMBA the most exciting development in the whole fast-moving field is the application to organoids of “single-cell transcriptomics”, a new technology that enables researchers to analyse individual neurons through the activity of their genes.
“This is already showing remarkable similarities between cells in an organoid and in a real human brain,” he says. “The insights we gain will turn around our view of the mechanism of some diseases.”
Lancaster and Knoblich believe brain organoids are ripe for commercialisation. They announced last month that they had co-founded a company in Vienna, called a:head, to do so. “Our cerebral organoids provide us with a fascinating new tool...to study neuropsychiatric disorders at a level of detail never possible before,” says Knoblich.
The new company will sell the technology for the pharmaceutical industry to test experimental drugs on mini-brains.
Complexity
The science may be advancing rapidly but researchers point out the huge gulf that still separates organoids and real human brains in terms of their size, organisation, complexity and ability to communicate with the outside world.
The first point is how small they are.
“An organoid lies somewhere between crayfish and zebrafish brains in size,” Lancaster says. “It has about one million neurons, compared with 10 million in a zebrafish, 70 million in a mouse and 86 billion in a human brain.”
Neurons in an organoid are electrically active, firing spontaneously like those in a human brain but usually in a disorganised way. Last month biologists at UC San Diego detected the first brain waves in organoids, resembling those in preterm babies, though the researchers said these were unlikely to be a sign of mental activity.
Unlike brains in live animals, they have no link with the external environment – and no researchers have announced plans to provide one – but it is possible to imagine a future project in which an organoid is given some form of sensory input or output via an electronic biochip.
To put the potential of organoids into context, Lancaster says they should be compared with other “brain surrogates” that scientists can use now or in the future to study the human brain without experimenting on living people.
Lab animals are the traditional human substitute, with mice and rats used in their millions by scientists around the world. But rising standards of animal welfare are circumscribing the types of experiments that can be carried out, particularly on primates, while biologists are coming to see that the neural difference between humans and other animals is greater than many had appreciated in the past.
“A human brain is not just an enlarged version of a mouse brain,” says Knoblich. “Its structure arises from fundamentally new mechanisms that do not exist in the rodent. That’s not to say that animal models are useless, but certain genetic mutations have a far stronger – or sometimes quite different – effect in humans than in mice.”
Two-dimensional cultures of neurons created from patients’ stem cells are proving invaluable for studying some neurological diseases. If a project requires a concentrated source of a particular cell type, such as motor neurons, then a 2D culture is likely to be the best option, but it is less useful than an organoid for studying overall brain development.
Living brain tissue removed from patients undergoing neurosurgery might be another option. But chimeras (animals containing cells from two different species) offer the most intriguing – and potentially worrying – prospect. Since well before last year’s implantation of a human organoid into a mouse at the Salk Institute, scientists have been creating chimeras with a substantial human neural component by injecting human stem cells into mouse embryos or the brains of mouse pups.
Chimeras
Mice with humanised brains are “going to be highly limited in what they can do” because of their small size and short lifespan, says Lancaster, who is not working on chimeras herself, but humanised pigs might pose a serious ethical problem. Researchers are working on human-pig chimeras to produce organs for transplantation that would be compatible with a patient’s immune system, she points out, and “in theory you could make a fully formed human brain in a pig”.
While Lancaster is happy to discuss the morality of different paths that neurotechnology may take – and the need to prevent unacceptable developments – she is confident that her own work offers huge potential for understanding the human brain in sickness and in health, with no short-term ethical downside.
As she replaces the organoid dish on its gyrating incubator shelf, it is remarkable to reflect that the white blobs are seething with electrical and biochemical activity. Analysing this neural ferment could help to explain why our human brains are so much more powerful – and more vulnerable to disease – than those of any other creature on the planet. – Copyright The Financial Times Limited 2019